1. Introduction
Strawberry is a crop sensitive to plague attack. To keep the crop healthy, several pesticides are currently applied. Strawberry is one of the fresh fruits with the greatest number of pesticide residues present among fruits and vegetables in the last years1. An average of between 7 and 8 pesticide residues per sample have been reported in different monitoring programs 2)(3) .
Mexico and the United States are the main exporters of strawberries4, and a lot of positive findings are reported in these countries. The U.S. Food and Drug Administration (FDA) carries out continuous monitoring and reports results annually to demonstrate that levels of pesticide residues in the U.S. food supply are well below established safety standards, yet they are consistently included in the “dirty dozen” for the number of pesticide residues found in fruit. Spain is the leading exporter of strawberries within the European Union (EU), while other countries such as Slovenia, Austria, and Germany also play significant roles in strawberry production. In the past four years, several warnings have been recorded in the Rapid Alert System for Food and Feed (RASFF) system, including cases of flonicamid and spinosad residues in strawberries from Spain and Germany, respectively, as well as multiple alerts for strawberries originating from Egypt5.
Meanwhile, China is the world's major strawberry producer. This country reports the monitoring of 242 strawberry samples from the local market in 2017-2018, detecting at least one pesticide residue in 26% of the samples, in some cases exceeding the Maximum Residue Level (MRL)6. In Brazil, Paim Fraga and others reported that 35 different active ingredients were detected in strawberry samples (2018-2019) summing up to a total of 303 detection events7. In Australia, a pilot study to monitor pesticide residues in fruit and vegetables revealed residues that exceeded maximum residue limits in strawberries8. As seen, the high number of pesticide residues in strawberries is a worldwide phenomenon.
In Uruguay, strawberries are mainly produced by family farmers. There are two principal areas of the country where strawberries are cultivated: in the south, the San Jose region, and in the north of the country, the area of Salto and Paysandú concentrates the greatest production. The technological package allowed for strawberry production in Uruguay is varied and vast. Some years ago, strawberry cropping was performed only in spring in open-field plantations. Nowadays, it is performed in greenhouses for more than 9 months a year, ensuring the fruit supply for longer periods9. Fruit availability changed the food habits of the population, driving a change in their pesticide exposure. Strawberry cultivation faces significant challenges from various diseases and pests, particularly fungi that cause fruit rot and leaf spots. These problems are highly destructive and often result in substantial crop losses, compelling farmers to rely heavily on pesticides for control 10)(11) . Furthermore, strawberries produce fruit gradually, requiring continuous and frequent harvesting, usually two to three times per week, depending on the season. This harvest strategy complicates pesticide management, as the short interval between harvests (one or two days) limits the use of pesticides, since very few have such short pre-harvest intervals 10)(12) .
In a previous work studying the safety of strawberries after minimal processing, Pequeño and others13 reported different residues in the analyzed raw fruit: on average, four pesticides per analyzed sample. These results highlighted the need to monitor pesticide residues content in strawberries for longer periods to assess their safe consumption. To start a pesticide risk assessment for this product, a monitoring program showing the baseline of pesticide residue occurrence in strawberries is needed. Such a monitoring program could give insights into the agricultural practices followed providing valuable information for regulatory and enforcement purposes, besides the already mentioned risk assessment studies. In addition, some strawberries have been exported, and new market possibilities have been opened9. Monitoring data will show the fitness of the Uruguayan strawberries to accomplish the requirements abroad.
The yearly monitoring of pesticide residues in strawberry production provides information for future risk assessment studies and checks Good Agricultural Practices' (GAPs) accomplishments. The data will show a first-hand overview of the situation for regulators for enforcement purposes and to farmers willing to export the product, helping to avoid commercial barriers.
2. Materials and methods
2.1 Source of fruit samples
Fifty-eight fresh strawberry samples were collected randomly from supermarkets and street markets in Paysandú and Montevideo, Uruguay. It was not possible to trace the fruit back to the producer. Each sample consisted of 1 kg of fruit collected randomly.
2.2 Pesticide analysis
All samples were analyzed for 41 pesticide residues and 13 pesticide metabolites. The pesticide scope was defined according to MGAP recommendations for strawberry production in Uruguay14 and from the international information on strawberries rejected by exceeding the MRLs5. The final studied pesticides and metabolites are shown in Table 1.
The methodology applied was the EU official method for pesticide residues QuEChERS citrate method EN 1566215 and validated following Document SANTE Guidelines 13)(16) .
Table 1: Mass spectrometry optimized parameters for parent pesticides and metabolites
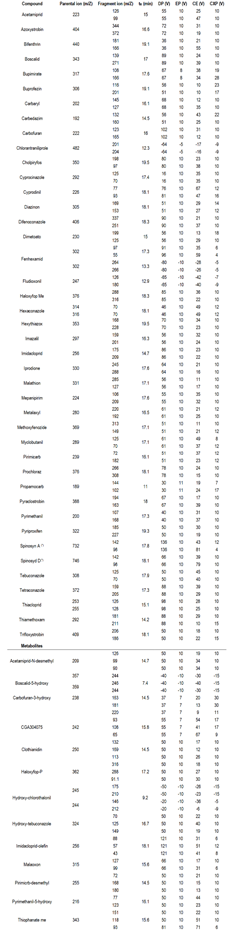
(*) Spinosad is reported as a sum of isomers, A and D, and their instrumental conditions are adjusted for each isomer, as required by the regulations.
2.2.1 Reagents and materials
The reference pesticide standards were ≥ 96% purity supplied by Sigma-Aldrich (Steinheim, Germany) and Dr. Ehrenstorfer GmbH (Augsburg, Germany). Stock solution standards were prepared individually between 1000 and 2000 µg mL-1 concentration in acetonitrile (MeCN) or ethyl acetate (EtAc) and stored in amber glass vials at -20 °C. To obtain the final working mix, appropriate dilutions of the stock solutions were prepared at 10 µg mL-1 in acetonitrile.
The solvents used for the extraction and chromatography step were methanol (MeOH) and MeCN, both HPLC-grade acquired from Carlo Erba (Italy). To enhance ionization, formic acid (HCOOH) 88% from Macron Chemicals (Pennsylvania, USA) and ammonium formate (CH5NO2) from Fluka Analytical (Seelze, Germany) were employed. A Thermo Scientific (Marietta, OH, USA) EASY0 pure RoDi Ultrapure water purification system generated the deionized water (18 MΩ). The salts used during the extraction, salting-out, and clean-up step include magnesium sulfate anhydrous (MgSO4) from Carlo Erba (Italy), sodium chloride from Dorwil (Bs. As., Argentina), di-sodium hydrogen citrate 1.5 hydrate (C6H6Na2O7·1.5H2O) from Scharlau (Barcelona, Spain), sodium citrate dihydrate (C6H5O7Na3·2H2O) from J.T. Baker (Phillipsburg, NJ, USA), and to create an in-situ buffer at pH: 5-5.5. For the clean-up step, primary and secondary amine from Chromabond® (Macherey-Nagel, Germany) was employed.
2.2.2 Strawberry primary sample conditioning
Both Codex Alimentarius and EU regulations establish that strawberries must be processed for pesticide residues analysis without the calyx 17)(18) . After calyx removal, strawberries were chopped using a mixer (Smart Life model SL-HB988) to obtain a homogenate following the guides. Afterward, three sub-samples of 10 g from each sample were extracted, cleaned up, and analyzed for pesticide and metabolite residues.
2.2.3 Sample preparation from the extraction step and instrumental determination
The sample preparation was performed employing the reported methodology by Pequeño and others, that had been validated within the laboratory conditions13. In this study, the scope of the method was extended to encompass additional relevant compounds (15 new active ingredients and 13 metabolites), and the validation was accomplished for the new scope (Table 2).
2.2.4 Instrumental pesticide determination of pesticide metabolites
Metabolites of pesticides in strawberries were analyzed using a Shimadzu SIL 20AT liquid chromatography system coupled to a triple quadrupole 3500 QQQ from Sciex used in MS-MS mode. The chromatographic column used was a Zorbax Eclipse XDB of C18 (150 × 4.6 mm x 3.5 µm). The mobile phase compositions were the same used by Pequeño and others13. The analysis was carried out in positive and negative ionization modes. In the positive acquisition, the mobile phase A consisted of 5 mM ammonium formate, in high ultra-purity water with addition of 2% of MeOH and 0.1% of HCOOH. Mobile phase B is 5 mM of ammonium formate in MeOH and uses 2% of H2O and 0.1% of HCOOH. The initial gradient for the positive ionization was 90:10 from A to B up to minute 8, then 100% of B to minute 15, and came back to the initial gradient at the final method, on minute 21. During ESI negative acquisition, mobile phases consisted of (A) 0.1% HCOOH in high ultra-purity water and (B) acetonitrile. The respective initial gradient was 70:30 from A to B up to minute 6, afterward 100% of B to minute 11, and came back to the initial gradient at the final method, on minute 16. For both experiments, the temperature of the source was settled at 500 °C. The ionization voltage was +5000 V and -4500 V for positive and negative ionization modes, respectively. The gas curtain was nitrogen at 20 psi and the nebulizer gas was air at 50 psi. The selected acquisition mode was Multiple Reaction Monitoring (MRM). For all the new analytes two transitions were optimized. All the mass spectrometry parameters and retention times are presented in Table 1. Analyst software v. 1.7 (Sciex, Massachusetts, USA) was used for data acquisition and data processing for qualitative and quantitative analyses.
2.3 Method validation
Sample extraction protocol was reported by Pequeño and others, and the validation was carried out for the selected analytes13. To assess the trueness and precision of the method for the extended scope, the experiment was conducted at three fortification levels (0.005, 0.01, and 0.05 mg kg-1) with five replicates for each level. The acceptance criteria were: mean recovery within 70-120% with an RSD ≤ 20%; repeatability and reproducibility were also assessed for all the studied analytes.
Reproducibility was evaluated by performing the method on three different days. Limit of quantification (LOQ) was the lowest concentration level with recoveries between 70-120% and RSD ≤ 20%. Matrix effects were also assessed by comparing the calibration curves in solvent and matrix.
3. Results
The validated method employed in a previous study was expanded from 26 to 41 compounds aligning with the crop protection active principles approved in the country for use in strawberries14.
Strawberry cultivation faces significant challenges from various diseases and pests, particularly fungi that cause fruit rot and leaf spots. These problems are highly destructive and often result in substantial crop losses, compelling farmers to rely heavily on pesticides for control 10)(11) . Furthermore, strawberries produce fruit gradually, requiring continuous and frequent harvesting usually two to three times per week, depending on the season. This harvest strategy complicates pesticide management, as the short interval between harvests (one or two days) limits the use of pesticides, since very few have such short pre-harvest intervals 10)(12) .
Regarding the safety of strawberries after minimal processing, different residues in the analyzed raw fruit were reported by Pequeño and others13: on average, four pesticides per analyzed sample. These results highlighted the need to monitor pesticide residue content in strawberries for longer periods to assess their safe consumption.
3.1 Scope expansion
The figures of merit for the validation of the incorporated parent compounds in the expanded scope, in accordance with SANTE 11312/2021 guidelines16, are presented in Table 2. Strawberry sample used as blank in trueness and calibration studies was previously checked for pesticide residues. Recoveries ranged from 82 to 120%, with RSD consistently below 23% for all compounds. Quantification involved five-level matrix-matched calibration curves, spanning a linearity range from 0.005 to 0.1 mg kg-1 and matrix effects were observed below 20%. A matrix matched calibration curve was used to quantify the detected residues. The final pesticide list and the LOQ are presented in Table 3. According to SANTE 11312/2021 guidelines, the limit of detection (LOD) of the method is the lowest validated recovery, thus it can be regarded as the LOQ. To further investigate the application history of the evaluated samples, instrument conditions following SANTE 11312/2021 guidelines (C45-C47)16 were adjusted for the screening of 13 pesticide metabolites included in the residue definition of the parent compounds.
Table 3: Pesticide, limit of quantitation (LOQ), range of concentration for positive detected in samples, MRLs and residue definition
Compound | LOQ (mg kg-1) | Codex MRL (mg kg-1) | EU MRL (mg kg-1) | Concentration range (mg kg-1) | Residue definition |
Acetamiprid* | 0.005 | 0.5 | 0.5 | 0.009-0.02 | Sum of acetamiprid and its desmethyl (IM-2-1) metabolite, expressed as acetamiprid. |
Azoxystrobin | 0.005 | 10 | 10 | 0.009-0.42 | Expressed as azoxystrobin. |
Bifenthrin | 0.005 | Not defined | 1 | ND | Bifenthrin (sum of isomers). |
Boscalid* | 0.010 | 3 | 6 | <LOQ-1.2 | Sum of boscalid and its hydroxy metabolite 2-chloro-N-(4′-chloro-5-hydroxybiphenyl-2-yl)nicotinamide (free and conjugated) expressed as boscalid. |
Bupirimate | 0.005 | Not defined | 1.5 | ND | Includes desethyl ethirimol, but the EU reference labs identified the reference standard for the desethyl ethirimol as commercially not available. |
Buprofezin | 0.005 | 3 | 0.01 | ND | Expressed as buprofezin. |
Carbaryl | 0.005 | Not defined | 0.0-1 | ND | Expressed as carbaryl. |
Carbendazim | 0.005 | Not defined | 0.1 | 0.006-2.5 | Sum of benomyl, carbendazim and thiophanate-methyl, expressed as carbendazim. |
Carbofuran | 0.010 | Not defined | 0.005 | ND | Sum of carbofuran (including any carbofuran generated from carbosulfan, benfuracarb or furathiocarb) and 3-OH carbofuran expressed as carbofuran. |
Chlorpyrifos* | 0.005 | Not defined | 0.01 | 0.007-0.04 | Expressed as chlorpyrifos |
Cyproconazole* | 0.005 | Not defined | 0.05 | 0.02-0.11 | Free cyproconazole and conjugated. |
Chlorantraniliprole | 0.005 | Not defined | 1 | <LOQ | Expressed as chlorantraniliprole. |
Cyprodinil | 0.005 | Not defined | 5 | 0.008-1.0 | Sum of cyprodinil and CGA 304075 (free and conjugated), expressed as cyprodinil. |
Diazinon* | 0.005 | 0.1 | 0.01 | 0.006 | Expressed as diazinon. |
Difenoconazole | 0.005 | 2 | 2 | 0.006-0.27 | Expressed as difenoconazole. |
Dimethoate | 0.005 | Not defined | 0.01 | ND | Dimethoate and omethoate (measured and reported separately). |
Fenhexamid | 0.005 | 10 | 10 | 0.007-1.0 | Expressed as fenhexamid. |
Fludioxonil | 0.005 | 3 | 4 | 0.005-0.16 | Sum of fludioxonil and metabolites determined as 2,2-difluorobenzo(1,1)dioxole-4-carboxylic acid, expressed as fludioxonil. |
Haloxyfop Me | 0.005 | Not defined | 0.01 | ND | Sum of haloxyfop, including haloxyfop-P, its salts and conjugates expressed as haloxyfop (sum of the R- and S- isomers at any ratio). |
Hexaconazole | 0.005 | Not defined | 0.01 | ND | Expressed as hexaconazole |
Hexythiazox* | 0.005 | 6 | 6 | 0.008-0.06 | Sum of hexythiazox and all metabolites containing the trans-5-(4-chlorophenyl)-4-methyl-2-oxothiazolidine-moiety (PT-1-3), expressed as hexythiazox. |
Imazalil* | 0.005 | Not defined | 2 | 0.008 | Free and conjugated imazalil, sum of imazalil and metabolite FK-772 (any ratio of constituent isomers), expressed as imazalil. |
Imidacloprid | 0.005 | 0.5 | 0.01 | 0.02 | Sum of imidacloprid and its metabolites containing the 6-chloropyridinyl moiety, expressed as imidacloprid. |
Iprodione | 0.010 | 10 | 0.01 | 0.01-0.71 | Sum of iprodione and all metabolites containing the 3,5-dichloroaniline moiety, expressed as iprodione. |
Malathion | 0.005 | 1 | 0.02 | ND | Sum of malathion and malaoxon expressed as malathion. |
Mepanipyrim | 0.005 | Not defined | 3 | ND | Expressed as mepanipyrim. |
Metalaxyl* | 0.005 | Not defined | 0.6 | 0.007-0.20 | Metalaxyl and metalaxyl M (sum of enantiomers) and N-(2-hydroxymethyl-6-methylphenyl)-N-(methoxyacetyl) alanine methyl ester (M8; free and conjugated; sum of enantiomers), expressed as metalaxyl. |
Methoxyfenozide* | 0.010 | 2 | 2 | 0.04 | Expressed as methoxyfenozide. |
Myclobutanil* | 0.005 | 0.8 | 1.5 | 0.006 | Sum of myclobutanil, α-(4-chlorophenyl)-α-(3-hydroxybutyl)-1H-1,2,4-triazole- 1-propanenitrile (RH-9090) and its conjugates, expressed as myclobutanil. |
Pirimicarb | 0.005 | Not defined | 1.5 | ND | Sum of pirimicarb, and its demethyl, demethylformamido metabolites, expressed as pirimicarb. |
Prochloraz* | 0.005 | Not defined | 0.03 | 0.02-0.07 | Sum of prochloraz and its metabolites containing the 2,4,6-trichlorphenol moiety, expressed as prochloraz. Includes also sum of prochloraz, BTS 44595 (M201-04) and BTS 44596 (M201-03), expressed as prochloraz. |
Propamocarb | 0.005 | Not defined | 0.01 | 0.03-5.3 | Sum of propamocarb and its salts N-oxide propamocarb; and N-desmethyl propamocarb, expressed as propamocarb. |
Pyraclostrobin* | 0.005 | 1.5 | 1.5 | 0.006-0.16 | Expressed as pyraclostrobin. |
Pyrimethanil | 0.010 | Not defined | 5 | 0.15 | Sum of pyrimethanil and 2-(4-hydroxyanilino)-4.6-dimethylpyrimidine, expressed as pyrimethanil. |
Pyriproxyfen* | 0.005 | Not defined | 0.05 | 0.08-0.1 | Expressed as pyriproxyfen. |
Spinosad | 0.005 | Not defined | 0.3 | 0.03 | Sum of spinosyn A and spinosyn D. |
Tebuconazole | 0.010 | Not defined | 0.02 | ND | Sum of tebuconazole, hydroxy-tebuconazole, and their conjugates, expressed as tebuconazole. |
Tetraconazole | 0.005 | Not defined | 0.15 | ND | Expressed as tetraconazole. |
Thiacloprid | 0.005 | Not defined | 1 | ND | Expressed as thiacloprid. |
Thiamethoxam | 0.010 | Not defined | 0.01 | ND | Thiamethoxam and clothianidin (considered separately). |
Trifloxystrobin | 0.005 | 1 | 1 | ND | Sum of trifloxystrobin and ((E,E)-methoxyimino-{2-(1-(3- trifluoromethylphenyl) ethylideneaminooxymethyl) phenyl}acetic acid) (CGA 321113), expressed as trifloxystrobin. |
ND: not detected. * Pesticides not allowed by the National Authority.
3.2 Monitoring results
3.2.1 Parent compounds
A total of 58 fresh strawberry samples were monitored. Results of the detection rate determined, and the EU and Codex MRLs for the evaluated pesticide residues are shown in Table 3. Pesticide residues were quantified in 56 of the different analyzed marketed strawberries. A total of 24 different pesticide residues were detected in the samples. Among these, 13 compounds showed a deviation of use as they are not permitted for strawberry crops in Uruguay (Table 3)14. Pesticide residue concentrations ranged between 0.005 and 5 mg kg-1. Of the 24 determined pesticides, 16 were fungicides and 8 were insecticides (Table 3). The five most frequently detected pesticides in the analyzed samples were fungicides: cyprodinil (71%), carbendazim (45%), azoxystrobin (36%), fludioxonil (33%), and boscalid (31%). Additionally, eight insecticides were quantified in the analyzed samples, with pyriproxyfen the most frequent one (9%). Noticeably, an average of four pesticide residues was found in those positive samples (Figure 1). From the evaluated samples, at least three pesticide residues were detected in 81% of them. Only 16% of the 56 samples contained 1 or 2 measurable pesticide residues, but no trend in the occurrence of any particular analyte was observed.
3.2.2 Pesticide metabolites analysis
Thirteen pesticide metabolites were screened in the targeted analysis (Table 1). The lowest identification level for pesticide metabolites was 0.001 mg kg-1. CGA304075, the main cyprodinil metabolite, was the only detected metabolite and was present in 61% of the samples containing cyprodinil (Figure 2). Due to its high occurrence, it should continue to be sought and measured using validated quantitative multi-residue methods. Further work shall be focused on the validation of the different metabolites.
Particularly, chlorothalonil-4-hydroxy, the main chlorothalonil metabolite, was incorporated into the screening method. Chlorothalonil has not been included in the validated method scope because it is a GC amenable compound. This fungicide is reportedly applied in strawberry crops. The investigation of the LC-amenable metabolite was conducted to assess the application of the parent compound. The screening of pesticide metabolites provides the possibility to extend the laboratory analytical scope to analytes which potentially have a low probability of being present in the samples16.
4. Discussion
Fruit monitoring surveys in different countries found similar results to this work 6)(7) 19. Regional and international reports have shown a high presence of fungicide residues in strawberries. In Brazil, the top 5 fungicides detected during 2018-2019 were procymidone, carbendazim, difenoconazole and azoxystrobin7. In Serbia, the analysis of 76 strawberry samples in 2019 revealed that 75% contained pesticide residues. The most frequently detected pesticides included pyrimethanil, azoxystrobin, fluopyram, acetamiprid, chlorpyrifos, boscalid and metolachlor, showing the presence of fungicides, insecticides, and herbicides in the fruit20. In this work, 96% of the samples had quantifiable pesticide residues. Of the positive ones, 36% were above their MRLs and 18 samples showed the presence of not authorized pesticide14.
In this work, cyprodinil and fludioxonil co-occurred in 26% of the total samples. This result is expected as commercial formulations containing a mixture of cyprodinil and fludioxonil are registered for use in strawberries in Uruguay14. A similar scenario was noted for pyraclostrobin and boscalid, which are commonly used in a combined formulation to treat graymold 10)(12) 14; in this case, the co-occurrence of both fungicides was 21%.
Regarding insecticides, the most frequently detected compound was pyriproxyfen in 9% of the samples, an insect juvenile hormone mimetic21 classified in the III WHO toxicity category22. Pyriproxyfen residues were also detected with low frequency (1.6%) in samples from Rio Grande do Sul strawberry production. However, the most frequent insecticide was thiamethoxam, present in 40.32% of the reports7.
These results are consistent with many worldwide reports indicating that strawberries are one of the most contaminated fruits 1)(3) . Compared with the reports list, high fungicide occurrence is generally observed in strawberry samples7. However, some studies also indicate that insecticides and herbicides are among the most frequently found pesticides 20)(23)24)(25) .
In Uruguay, Resolution No. 75/018 from the General Directorate of Agricultural Services of the Ministry of Livestock, Agriculture and Fishery25 stipulates that when there are no MRLs established for a pesticide by national regulations or the Codex Alimentarius, MRLs or tolerances from the European Community (EU) or the competent authority of the United States will apply. The Codex Alimentarius26 and EU MRLs18 for the studied pesticides in the present work are shown in Table 3. It is important to notice from this survey results that international MRLs were exceeded for 7 pesticides: carbendazim, chlorpyrifos, cyproconazole, iprodione, prochloraz, propamocarb and pyriproxyfen (Figure 3). Except for iprodione, the other six pesticides that exceeded EU MRLs do not have defined MRLs in Codex Alimentarius 18)(26) . The presence of chlorpyrifos, cyproconazole, prochloraz and pyriproxyfen indicates improper use. The presence of unauthorized pesticide residues not only points to illegal use but also reflects irresponsible practices. Since these pesticides are not registered for strawberries, there are no Good Agricultural practices to follow. The absence of data on post-harvest intervals (PHI) implies a risk for consumers. Moreover, the findings indicate a lack of awareness among farmers about the risks associated with pesticide application.
In the case of pyriproxyfen, propamocarb, and iprodione, 100% of the samples containing them exceed the MRL. Regarding iprodione, it is crucial to note that it exceeded the EU MRL27 set at 0.01 mg kg-1, but it did not exceed the Codex MRL, which is established at 10 mg kg -1(26) . This highlights a gray area between international regulations where the established limits contradictorily differ by three orders of magnitude.
In Uruguay, there is no comprehensive program in place to ensure a high level of consumer protection through the monitoring of pesticide residues in harvested horticultural products. The few analyses that are conducted are insufficient, and there are no penalties for products exceeding maximum residue limits. Addressing this issue requires a multidisciplinary and multi-institutional approach, including promoting technical guidance, strengthening monitoring capacities for pesticide residues, and making the results publicly available.
5. Conclusions
The present study indicates the presence of multiple pesticide residues on strawberries. The situation highlighted in this study is significantly more alarming than what has been reported globally. In some instances, up to six pesticides were detected in a single sample. This initial data collection serves as a crucial step to be used in risk assessment studies and verify GAPs' accomplishments. Data will provide a first-hand overview of the situation for regulators for enforcement purposes and for those farmers willing to export the product, helping to avoid commercial barriers. This will help to strength and standardize farmers’ understanding of strawberry pest-control measures and provide a scientific basis for the government to conduct quality, safety, and risk management of strawberry products.